What Is Vibration Analysis?
Vibration analysis is the practice of monitoring and assessing machinery’s vibration characteristics to identify anomalies and evaluate equipment health. By measuring variations in amplitude, intensity, and frequency, this technique allows for detecting changes in the vibration signature that can indicate underlying physical conditions.
An accelerometer captures the vibration data, converting it into voltage signals representing the frequency and magnitude of the machine’s movements. This information is essential for diagnosing potential issues, predicting failures, and maintaining optimal machine performance.
Data collected from the accelerometer is fed directly into a data collector, which records the signal as either amplitude versus time (time waveform), amplitude versus frequency (fast Fourier transform), or both.
The next step involves using advanced computer algorithms to process this data, which is then further analyzed by engineers or trained vibration analysts. This analysis helps determine the machine’s health and identify potential issues such as looseness, imbalance, misalignment, lubrication problems, and other impending faults.
Vibration analysis can be applied to:
- Identify developing issues that can be repaired to extend the machine’s lifespan.
- Detect and track chronic problems that are beyond repair and will progressively worsen.
- Set acceptance testing standards to verify the proper execution of installations and repairs.
- Enable 24/7 continuous vibration monitoring to predict failures as part of a predictive maintenance strategy.
What Does Vibration Analysis Detect?
Vibration analysis is a powerful tool for assessing equipment condition and performance. Maintenance teams can identify issues that compromise machine reliability by analyzing vibration data. For example, it can detect broken welds or bolts, evaluate the integrity of rotor bars in motors, and check for non-concentric air gaps between rotors and stators.
Additionally, vibration analysis can reveal structural looseness, rotating component looseness, and harmful resonance.
This technique is beneficial for verifying the proper installation of bearings, such as ensuring that taper bearings have adequate clearance between the rotating element and the outer race. Misalignments in assemblies, such as pumps, can also be detected, allowing teams to identify issues like binding rotating elements, uneven bases, or torsion between connected components.
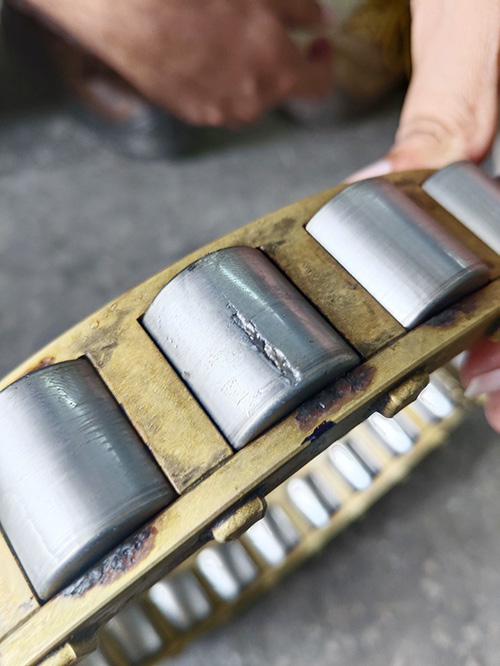
Bearing failure detected with vibration analysis when the waveform Pk-Pk trend increased sharply.
How Does Vibration Analysis Detect Possible Failures?
Understanding how vibration analysis predicts potential asset failures is crucial to effective machinery maintenance. Every machine produces a unique vibration signature or profile during operation. Monitoring and analyzing these signatures can identify deviations or anomalies, signaling potential issues within the equipment or its components.
When an unusual vibration pattern is detected, maintenance teams assess whether it indicates a failure. Spectrum analysis is vital in this process using techniques like the Fast Fourier Transform (FFT). By converting the vibration data from the time domain to the frequency domain, spectrum analysis reveals the specific frequencies within the vibration signal. These frequencies help pinpoint the exact source of the anomaly, allowing for accurate diagnosis and timely intervention to maintain the machine’s health and functionality.
The Fundamentals of Vibrations
Vibrations are complex and require consideration of multiple parameters for accurate analysis. The main parameters include amplitude, frequency, and phase:
- Amplitude refers to the size or magnitude of the vibration, measured in units such as displacement (mils or micrometers), velocity (inches per second or millimeters per second), or acceleration (g’s).
- Frequency indicates how many oscillations occur within a specific time frame, typically measured in Hertz (Hz).
- Phase represents the timing relationship of the vibration waveform, usually measured in degrees.
Vibration analyzers utilize these parameters, waveform shape, and harmonic content to diagnose and assess the severity of specific machine faults.
Vibrations generally fall into two categories:
- Forced Vibration: Caused by an external force, such as the vibrations generated by an operating motor or pump.
- Natural Vibration (Resonance): Occurs when a system vibrates at its natural frequency. This can be problematic if the natural frequency aligns with the operating frequency, leading to excessive and potentially damaging vibrations.
How Is Vibration Measured?
Vibration is measured using a variety of sensors designed to capture different aspects of vibration, such as displacement, velocity, and acceleration. These sensors employ various technologies, including piezoelectric (PZT) sensors, microelectromechanical systems (MEMS), proximity probes, and laser Doppler vibrometers.
PZT sensors are the most commonly used. They generate voltage when they are deformed by vibration. This voltage is then digitized and analyzed to represent the vibration characteristics. When selecting the appropriate sensor, factors such as vibration levels, dynamic range, maximum frequency range, and environmental conditions (e.g., temperature, humidity, and pH) must be taken into account.
Proper sensor installation is crucial for accurate data collection. The best practice is to stud mount the sensor on a flat, clean machine surface to capture a wide and smooth frequency spectrum.
If stud mounting isn’t possible, alternatives like magnet holders, wax, or adhesive can be used, with consideration given to the vibration levels and frequencies involved.
Typically, vibration signals fall below 20 kHz, although certain resonances may exceed this range. It is essential to carefully select the sampling rate to ensure the frequency range of interest is captured. Additionally, the recording duration should encompass several cycles of the machinery’s lowest operating speed to ensure comprehensive data collection.
Four Types of Vibration Analysis Equipment
Effective vibration testing requires precise measurement of vibration waves. There are four primary methods for measuring these waves:
Global Level Vibration Meters
These meters, with or without filters, assess a machine’s or structure’s overall vibration level. Filters allow the selection of specific frequency ranges, helping to isolate particular issues.
Accelerometer
These devices measure the acceleration of a vibrating object, providing insights into the amplitude and frequency of the vibration.
Velocity Sensors
These sensors help determine the amplitude, frequency, and velocity of vibration by measuring the velocity of a vibrating object.
Displacement Sensors
These sensors track the displacement of a vibrating object, offering a measurement of the vibration’s amplitude.
Where Should Vibration Sensors Be Installed?
It’s recommended that vibration sensors be placed on your most critical machinery. These sensors are essential for monitoring the condition of all your rotating equipment, such as:
- Motors
- Gearboxes
- Pumps
- Fans and belts
- Conveyor systems
- Automated assembly lines
- Chillers
When beginning a condition monitoring program, prioritize installing vibration sensors on crucial equipment for sustaining production. Position the sensors close to the motor, pump, and shaft bearings. If you’re uncertain about the best locations or installation methods, consult with condition monitoring specialists.
Approaches to Vibration Analysis
While accelerometers remain the most common tools for collecting vibration data, advancements in sensor technology have introduced non-contact, high-speed laser sensors capable of detecting issues that traditional accelerometers might miss.
These innovations provide more accurate, localized analysis and expand the methodologies available for vibration analysis. Vibration analysis is typically categorized into four fundamental principles, each offering specific insights into the operating conditions and characteristics of the vibrating components.
- Time Domain: When a vibration signal from a transducer (a device that converts a physical quantity into an electrical signal) is displayed as a waveform on an oscilloscope, it represents the time domain, where the amplitude is plotted against time. While spectrum analysis often identifies most machine vibration issues, specific problems are more effectively detected through time-domain analysis.
- Frequency Domain: When a waveform undergoes spectrum analysis, the result is a spectrum showing frequency versus amplitude, representing the frequency domain. The most detailed analysis of machinery vibration is conducted in the frequency domain using spectrum analysis.
- Joint Domain: Vibration signals change over time, so calculating multiple spectra simultaneously can provide valuable insights. The Gabor-Wigner-Wavelet technique, a joint time method, is used for this purpose. It calculates the fast Fourier transform (FFT) variations, including the short-time Fourier transform (STFT).
- Modal Analysis: This method involves taking measured frequency response functions of machinery and inputting them into a computer model. The model can animate different vibration modes, allowing engineers to simulate changes by adjusting factors like mass or stiffness to observe their effects on vibration behavior.
Understanding Acceleration, Velocity, and Displacement Parameters
The three key parameters commonly used in vibration measurement and analysis are acceleration, velocity, and displacement.
Acceleration is highly sensitive to changes in machine conditions and is often the most effective parameter for early problem detection.
Velocity is also valuable, particularly for identifying resonance conditions.
Displacement, though less sensitive to condition changes, is frequently used to measure vibration amplitude, which can indicate loose or damaged components.
The optimal parameter depends on the specific application, but generally, measuring all three provides a comprehensive understanding of the asset’s condition.
Benefits of Vibration Monitoring
Vibration monitoring, whether part of a predictive maintenance program or through continuous observation, offers significant advantages for equipment management and operational efficiency.
Lower Maintenance Costs
Vibration monitoring helps avoid expensive repairs and replacements by proactively addressing potential failures. This saves money and contributes to sustainability by reducing energy consumption.
Early Fault Detection
Advanced vibration analysis can detect issues before they become severe enough to be noticed by other means. This early detection allows maintenance teams to address problems before they lead to costly downtime.
Improved Maintenance Planning
With detailed insights into machinery condition, maintenance teams can better plan and schedule their work. This optimizes resource allocation, enhances equipment reliability, and reduces unplanned downtime.
Enhanced Safety
Vibration monitoring helps predict breakdowns and identify malfunctioning machinery, reducing the risk of accidents and injuries.
Optimized Machinery Performance
Continuous vibration monitoring ensures real-time data collection and analysis, allowing immediate response to detected anomalies. This is especially beneficial for critical, heavily used, or hard-to-access equipment, where early detection of issues can prevent major disruptions and financial losses.
By integrating these monitoring practices, organizations can maintain high levels of equipment reliability, minimize operational interruptions, and ensure a safer working environment.
The Role of Vibration Analysis in Predictive Maintenance
Predictive maintenance is a forward-thinking approach that focuses on performing maintenance only when necessary—specifically when there are clear indicators of imminent failure. Vibration analysis is a cornerstone of this strategy; continuously monitoring vibration patterns provides critical insights into the machinery’s condition.
Unlike preventive maintenance, which schedules repairs at fixed intervals regardless of the machine’s condition, predictive maintenance leverages real-time data from vibration analysis to assess when a machine is nearing failure.
This approach allows maintenance actions to be scheduled during planned downtimes, reducing the risk of unexpected breakdowns and optimizing the use of resources.
Vibration analysis has the ability to detect subtle changes in a machine’s vibration signature, such as those caused by misalignment, imbalance, or bearing wear. These early warnings let maintenance teams intervene before minor issues escalate into major failures. For instance, an increased vibration amplitude at a specific frequency might indicate that a bearing is beginning to wear out. By addressing this issue proactively, teams can prevent costly unscheduled shutdowns and extend the lifespan of the equipment.
Vibration analysis within a predictive maintenance framework can identify when a repair is needed and the root cause of the issue. Engineers can tailor their maintenance actions more precisely by distinguishing between various vibration sources—such as overall machine condition and specific faults like unbalance or looseness.
Incorporating vibration analysis into a predictive maintenance program offers a comprehensive, data-driven method for maintaining machinery health, helping ensure operational efficiency, and minimizing downtime.
Thank you Muhammad Waqas for the bearing failure image.