Overall Vibration Effectiveness (OVE) is a leading maintenance metric that measures the proactive management of vibration-related factors impacting machine reliability.
It evaluates the percentage of machines that meet defined targets for mechanical balance, alignment, and looseness or resonance management. By addressing these root causes of destructive vibration, OVE promotes longer equipment life, reduces downtime, and improves operational efficiency.
Along with its companion leading metrics shown in the figure above, OVE is intended to drive proactive behaviors that ensure plant equipment runs free of the destructive vibrations that occur when a machine is unbalanced, misaligned, not tightened properly, and/or operating at a resonant frequency.
The OVE metric intentionally excludes the predictive aspects of vibration-analysis-based predictive condition-monitoring activities intended to detect defective or worn-out bearings and other machine components that must be replaced or repaired.
Instead, OVE supports the management of essential root causes of failure, which, in turn, extends equipment life and averts failure. Overall Vibration Effectiveness drives up Mean-Time-Between/To-Failure (MTBF/MTTF), which is our goal.
What OVE Is and Why It’s Important
Vibration is a destructive dynamic force that compromises the dynamic clearances of bearings, gears, and other machine components. This leads to contact fatigue, abrasion, and adhesive wear, leading to failure. It also results in cyclic fatigue that results in the failure of bolts and structural elements.
Each component responds to vibration differently. As an example rule of thumb, though, reducing the overall vibration of rolling element bearings by half results in an eight times life extension (Berry, 1995). That means even small improvements to reduce vibration can dramatically affect machine life, reliability, and life-cycle cost of ownership.
Reducing overall vibration by half can extend bearing life by up to eight times.
We generally refer to vibration in terms of amplitude and frequency. Amplitude describes how much the machine is vibrating and is typically expressed as acceleration (mm/sec2 or in/sec2), velocity (mm/s or in/sec and/or displacement (mm or in). The frequencies at which the machine is vibrating can reveal the nature of the problem or the forcing function that’s creating the vibration.
Frequency is typically expressed as cycles per minute or revolutions per minute (CPM/RPM), cycle per second, or Hertz (CPS/Hz). We also routinely refer to vibration in terms of multiples of running speed or multiples of gear mesh frequency (GMF).
OVE is calculated by multiplying the following three factors:
- Percentage of machines that are mechanically balanced to defined targets.
- Percentage of machines that are mechanically aligned to specified targets.
- Percentage of machines that are free of mechanical looseness or resonance.
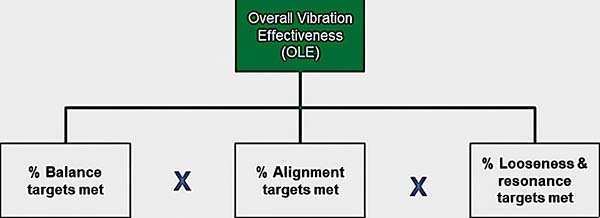
The simple formula for OVE, which is the product of multiplying the ‘behavioral inventories’ for the three input factors.
As with the other proactive leading metrics, consider each of the three OVE components/metrics an inventory process. We’re simply adding up the complying events and dividing by the total.
1. Percentage of Mechanical-Balance Targets Met
To operate reliably, rotating equipment, such as pumps, fans, motors, etc., must have an even distribution of mass around the machine’s rotation center.
A “heavy spot” results in relative centrifugal force (RCF), a dynamic force that is measurable as vibration at one-time (1X) the shaft’s running speed. Thus, an unbalanced rotor turning at 3,600 rpm would reveal a vibration at 3,600 CPM or 60 Hz. The dynamic unbalance force is linearly related to the mass of the unbalance and its distance from the center of the rotor.
Speed has a multiplicative effect on RCF and its associated vibratory forces. Assuming the same unbalance mass and distance from the rotation center, a machine turning at 3,600 rpm would produce four times as much RCF as the same machine turning at 1,800 rpm and 16 times more force than the same machine turning at 900 rpm.
An unbalanced rotor at 3,600 RPM exerts four times the force of one at 1,800 RPM.
The ISO 21940 standard provides the acceptance standards for precision balancing of rotors using a value known as the “G-unit.” The G-unit refers to the machine’s residual vibration at running speed. We use peak-observed vibration in mm/s at one-times (1X) running speed as an approximation in the field.
For example, 2.5 mm/s is a field approximation of a G = 2.5 in a shop balance environment on a rubber pad. However, be aware that other forcing functions can drive the 1X vibration. For critical systems, we like to balance to a G=1.0 or better. The API 610 standard for turbomachinery in the petrochemical industry calls for a G=0.70 (on the ISO scale) or better.
I like to see a G < 2.5 for important balance of plant equipment. The one-times running speed is the machine’s dominant vibration frequency, and it contributes a great deal to the overall vibration, which we employ for calculating life-extension opportunities.
To determine the percentage of balance targets met, simply divide the total number of machines operating at or below their specified maximum G-value and divide by the total number of machines being evaluated. Be sure to require that new equipment be precisely balanced as a part of your acceptance criteria.
When unbalance is observed in a piece of operating equipment, it’s crucial to quickly field balance or swap it out to be balanced in the shop. Unbalance is such a destructive force, especially for high-speed equipment, that it is dangerous to ignore it.
2. Percentage of Mechanical-Alignment Targets Met
Misalignment is another major source of destructive vibration. Centerlines of the shafts of driving and driven components must be free of any angular or offset misalignment, which introduces a wobble that can badly wear bearings, gears, and other components. Misalignment also creates cyclic forces that can lead to fatigue and even a shaft fracture.
Because misalignment produces a wobble, we usually identify it as a vibration peak at two-times a machine’s running speed that has higher amplitude than the one-times running speed peak.
So, for a machine that runs at 3,600 rpm, a peak at 7,200 cpm, or 120 Hz, would typically signify a shaft misalignment. We may also see an increase of vibration at three times the running speed (10,800 cpm, or 180 Hz in our example).
Misalignment creates a wobble that accelerates wear and leads to premature failure.
Misalignment in gears is trickier to detect. You must first calculate the gear-mesh frequency (GMF). This is straightforward for a single-reduction gearbox: You multiply the input speed by the number of teeth on the pinion.
So, for a gearset with an input speed of 900 rpm and a pion with 19 teeth, the GMF would be 17,100 cpm, or 285 Hz. If the amplitude of two times the GMF (34,200 cpm or 570 Hz) exceeds the amplitude of the GMF, you may have a gear-misalignment problem. If the gearset is a multiple-reduction unit, you’ll need to do the math to determine each GMF based on each pinion’s speeds and tooth counts.
For V-belts and synchronized belts, misalignment causes an increase in both the one-times and two-times running speeds. Note that there are other industry-specific alignment applications, such as conveyor belts in mining operations. Include them in your percent compliance for alignment calculations as applicable.
Computing the alignment component of OVE isn’t difficult: Divide the number of machines compliant with alignment targets by the total number of machines being monitored. Attend to misalignment issues promptly.
Fortunately, precision alignment is easier than it used to be, thanks to technological advances. Among them, David Zdrojewski created the “verti-zonta” compound alignment process utilizing true position sensing (TPS), so you’re aligning to true shaft centerlines.
It’s much easier than aligning rims and faces. Laser alignment and dial apps, in particular, can make alignment a breeze. For example, inexpensive laser pulley-alignment tools greatly simplify the alignment of belt-drive pulleys. (I like the ones that align to the center grooves of the pulleys instead of the shoulders.)
Remember to address runout and soft foot before attempting precision alignment, and always consider thermal growth.
3. Percentage of Mechanical-Looseness and Resonance-Management Targets Met
In my regular job as a consulting reliability engineer, it’s a toss-up as to which is the bigger problem in a plant: lubrication or loose fasteners. If machines are loose, they move around, vibrate, and shake themselves apart. We’re talking about the nuts and bolts of mechanical reliability here.
There are many different types of fasteners at a typical plant. Nuts, bolts, machine screws, rivets, and nails are temporary fasteners. Hoses and pipes have a variety of connector points. Weldments are common permanent fasteners in the plant.
Additionally, we must consider internal looseness. I typically focus on nuts and bolts, bearing and component fits, pipe and hose fasteners, and weldment quality. Nuts and bolts usually represent the largest part of that focus.
Loose fasteners and resonance are silent destroyers—addressing them is key to reliability.
To ensure secure bolted connections, we must have the right size of fastener (width and length); the right ISO or SAE grade of fasteners; the right fastener lubricant to reduce friction; the right tools (e.g., calibrated torque wrenches); and the right tightening technique (torque and sequence).
Upon inspection, a threaded bolt connection such as mounting a machine to its bases, the flange on a pipe, etc., should exhibit a) no missing or broken nuts or bolts; b) no evidence of self-loosing; c) no short-bolting (2-3 turns of the bolt should extend beyond the end of the nut); d) no incorrect washer usage (e.g., improper size, helical lock-washer for > 5/16″ of M8 fasteners); and e) no grade problems (I prefer SAE 5/ISO 8.8 or better unless the bolt is intended to shear).
Tightness can be checked with a properly calibrated torque wrench or preferably with an ultrasonic bolt tensiometer that accurately measures the elongation of a bolt, which creates the preload. Finally, vibration analysis can reveal structural or machine looseness by increased vibration at multiples of running speed ranging from two to 10 orders (and sometimes vibration at half-harmonics).
Loose bearing fit-ups can usually be detected by taking phase vibration readings every 30 degrees around the circumference of the bearing. If the bearing is internally loose, the frequency spectrum will look different at each measurement point. Welds can be visually inspected for quality and to check for cracking.
Simple weld-measurement tools are available to measure, among other things, leg length, cap reinforcement height on butt welds, and convexity or concavity. Non-destructive testing (NDT), ranging from simple dye penetrant analysis to sophisticated radiography, offers more robust weld-quality analysis.
Resonance is a condition where the machine is excited by an external or operating vibration at one or more of the machine’s natural frequencies. This results in an amplification of the destructive vibratory forces. Employ a bump test to determine your machine’s natural frequencies if they’re unknown to you.
If you must run at a speed that excites one or more of the machine’s natural frequencies, you’ll be required to add mass or stiffness such that the offending natural frequency is changed and resonance is avoided.
To determine the percentage of machines compliant with mechanical looseness and resonance management, divide the number of inspection points with no observed problems by the total number of inspection points.
Addressing root causes of vibration increases Mean Time Between Failures and reduces costs.
Proactively managing vibration in our machines is imperative for reliable, safe, and environmentally responsible operation. Start measuring, trending, and improving your Overall Vibration Effectiveness (OVE), and watch your MTBF/MTTF increase and your life-cycle cost of ownership decrease.