Precision maintenance requires a combination of skills, practical work instructions and methods, correct and properly calibrated tools, and the right parts in the right condition. Yet, parts are always on the list of usual suspects when conducting failure investigations and root-cause analysis (RCA).
Were the parts properly selected and specified when the machine was designed? Were the correct replacement parts purchased from quality suppliers? Were the parts correctly defined in the maintenance plan bill of materials (BOM)? Were the correct parts and materials pulled when kitting the job? Were the parts stored and picked correctly to avoid shelf degradation? This week’s article focuses on the design aspects of parts quality.
Design = The DNA of Machines
Design represents the genetic code or “DNA” of any electromechanical machine. For humans, DNA plays a huge role in determining one’s susceptibility to illness and disease. If your parents and grandparents had cancer, heart disease, or other affliction, you’re at greater risk for those disorders and must manage your lifestyle and medical care accordingly.
When designing equipment, we must consider static loads, dynamic loads, load excursions, and environmental conditions to which the equipment and its various parts will be subjected.
Design represents the DNA of a machine and determines its maximum reliability.
Static loads refer to the forces when the machine is in a stationary condition and are relatively easy to calculate. Dynamic loads refer to the forces when the machine is rotating, reciprocating, or otherwise moving. Load excursions occur when unforeseen static or dynamic forces are applied. Reliability engineers refer to this analysis as stress vs. strength interference.
Understanding Stress Vs. Strength Interference: Reliable Design
Strength of design, which refers to the strength of the materials and design as a whole, is usually represented as a bell curve to account for predicted variation in material strength. Stress refers to expected static and dynamic loads. It, too, is represented as a bell curve to reflect expected variations in stress, including anticipated excursions. Interference is where the tails of the two bell curves overlap.
Keeping the math simple and in generic units, if the material strength has an expected value of 150, with a standard deviation of 10, we could reasonably expect that in 99% of cases, the strength of the material will exceed 126.74, which is the average minus 2.3263 standard deviations.
Likewise, if the stress on the machine is 100. with a standard deviation of 10, we expect that in 99% of cases, the applied stress will be less than 123.26, which is the average plus 2.3263. Because the maximum applied stress of 123.26 is less than the minimum design strength of 126.74, we have no interference and can assume a reliable design (Figure 1).
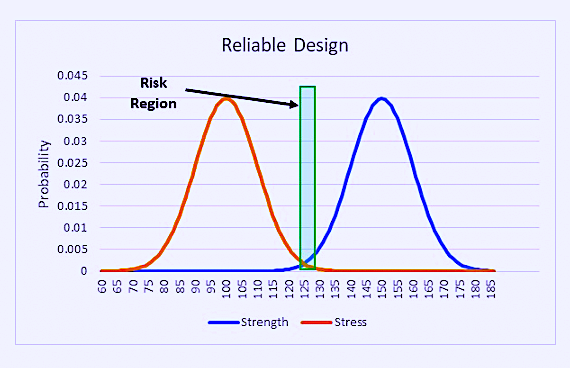
Figure 1: In a reliable design at the 99%-confidence level, there is no overlap between the bell curves for the applied stress and the material strength, so there is no interference.
Understanding Stress Vs. Strength Interference: Unreliable Design
What happens if our supply chain buys parts from a less-than-reputable source that lowers the average material strength from 150 to 140 and increases the standard deviation from 10 to 15?
Now, we expect the material strength to exceed 105.11 at the 99%-confidence level, down significantly from the design value of 126.74. Additionally, what if our operators push the equipment a bit too hard, which increases the average stress to 110, and we have some unexpected but transient load excursion that doubles the standard deviation from 10 to 20?
Now, we have a maximum expected load of 156.53. Under this scenario, there is a massive opportunity for the applied stress to exceed the material strength of the asset; there is substantial stress vs. strength interference, and we can expect reliability to suffer.
The increased standard deviation values flatten the curve and widen the distribution of expected stress and material strength (Figure 2).
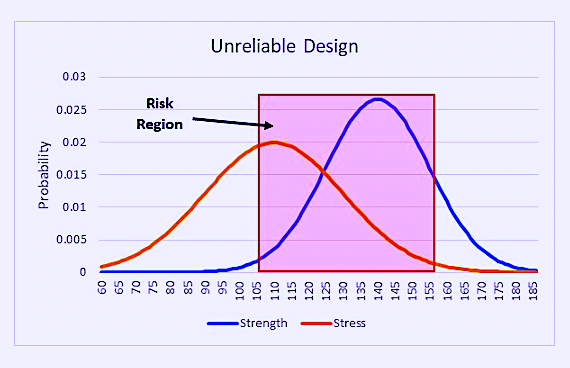
Figure 2. In an unreliable design, the tails of the two bell curves for the applied stress and material strength overlap and interfere significantly at the 99%-confidence level mark.
When the tails of stress and strength curves overlap, reliability suffers.
Equipment and process reliability requires precision in the form of materials selection, material-quality management, and installation. Design represents the DNA of a machine and determines the inherent maximum reliability the asset can achieve, regardless of how good we are at maintenance.
Understanding the static and dynamic loads that can be expected in an application and likely excursions defines the probability distribution of applied stresses.
Evaluating the strength and expected variation defines the probability distribution for design strength. Evaluating the probability that the applied stress curve will interfere with the material strength curve is an essential first step in our quest for precision and asset reliability.
Once parts and materials are properly selected, they must be shipped, stored, and staged to prevent damage and shelf degradation.
Rebuild For Reliability
Figure 3 illustrates the process flow I recommend for managing rebuilt parts and components in a plant:
The process of managing rebuilt parts and components has three basic elements. We must:
- Handle the failed items.
- Complete the rebuild of these items.
- Of course, manage the rebuilt items effectively.
Failure to apply precision at any stage in the process compromises the reliability of the parts and components, which, by extension, compromises the reliability of our assets and production processes. Let’s explore each of these elements in more detail.
Handling Failed Parts/Components
I prefer to geographically separate the handling of failed parts and components from the actual rebuild processes. Handing failed parts and components is a dirty process, whereas rebuilding them is, or should be, a clean process.
Too often, I see the disassembly and reassembly conducted in the same workshop, with no buffers or barriers. This allows dirt, dust, and grime to waft from dirty parts and components to clean ones. This type of situation compromises our precision goal of contamination control. Thus, you should set aside an area for the dirty operations of the rebuild process.
Separating dirty and clean processes is critical for contamination control.
Once a failed part or component is received, we must decide if it’s a suitable candidate for rebuild. If not, send it to the boneyard or, even better, stage the material to be sold as scrap and picked up by your contracted scrap handler.
If the component is deemed suitable for rebuild, decide whether the job will be done onsite or by an outside contractor. For example, some organizations will rebuild electric motors onsite if the job is limited to bearing replacement but contract the job out if a rewind is required.
Suppose the decision is to outsource the rebuild job. In that case, it’s appropriate to conduct a good external cleaning of the machine and stage it for pick up in an area that protects the item from further degradation.
If the item is being rebuilt in-house, conduct a thorough cleaning and prioritize and stage the parts to move to the cleanroom rebuild shop. Some disassembly and precleaning on the dirty side of the process is typically required before a part or component moves over to the cleanroom rebuild shop.
Be sure to stage those parts and components in areas free of vibration and sources of contamination to prevent further degradation, and then send any discarded parts to scrap.
Performing Precision Cleanroom Assembly
Several years ago, I worked with a client with a large population of Goulds 3196 pumps. Failure data analysis revealed those purchased new lasted much longer than those rebuilt onsite. It all came down to precision, though.
The rebuild shop was filthy. Rebuild instructions didn’t specify methods for precision bearing fit-ups. Bills of materials (BOMs) for the rebuilds didn’t exist. Threaded-fastener size, grade, torque values, and required thread lubricant weren’t specified. Pumps going through the rebuild process were not dynamically balanced.
Flushing and other lubrication practices weren’t specified or executed. In essence, the pump-rebuild process lacked precision and cost the company a lot of money. Here’s a simple checklist to support a cleanroom precision rebuild:
- Safety and job risk analysis (JRA)
- Correct bill of materials
- Required tools for precision rebuild
- Documented repair/rebuild procedures
- Drawings, images, P&ID, etc.
- Fit, tolerance, quantity, and quality details in instructions
- Focus on precision with FLAB:
- Fasteners
- Lubrication, including flushing
- Alignment
- Balance
- Post-rebuild testing, acceptance criteria, and quality assurance requirements
- Return to inventory and precision storage (if not immediately returned to service).
By separating the dirty and clean parts of the process, creating a cleanroom assembly environment, and implementing the precision fastener, lubrication, alignment, and balance (FLAB) practices, the company in question significantly improved the life and reliability of its rebuilt pumps.
The effectiveness of these practices isn’t limited to pumps, however. You’ll see similar results for gearboxes, fans, electric motors, blowers, and other critical equipment assets.
Managing Rebuilt Parts/Components
Here’s where everything comes together. Once you’ve completed a precision rebuild in a cleanroom environment and conducted any performance tests, transfer the rebuilt parts to the main warehouse, which must also be kept clean, temperature-controlled, and vibration-free to prevent shelf degradation.
I prefer to minimize the geographical distance between the failed parts handling area, the cleanroom precision parts and components rebuild shop area, and the general warehouse to reduce logistical issues and transfer costs.
Precision rebuild practices aren’t hard work—they’re smart work with big ROI.
The warehouse will be the source of new parts and materials required for precision rebuild. The cleanroom precision rebuild shop will feed rebuilt components that are ready for service into the warehouse.
In all aspects of the process, observe the 5S Principles of Sort, Sequence, Shine, Standardize and Sustain, First-in, First-out (FIFO) inventory practices, and other storeroom and material-handling best practices. Additionally, precision practices for components that outside contractors rebuild should be enforced.
We depend on countless rebuilt parts and components to deliver process reliability in our plants and facilities. Unfortunately, it’s rare to see personnel actually following precision-rebuild practices at sites.
Implementing the practices I’ve suggested here takes some work. But it’s not hard work. And it’s certainly not mysterious work. Most important, though, is the return on investment, which can be very impressive.
Initially published in The RAM Review.
Drew Troyer is a seasoned expert with over 30 years of experience in sustainable manufacturing, physical asset management, energy management, and reliability engineering. He has a proven track record of helping companies in the mining, resource, process, and manufacturing industries optimize their operations to be more sustainable, reliable, and profitable. Drew is a thought leader and a prolific author, with over 350 published works and extensive experience as a keynote speaker at global conferences. He is also a Certified Reliability Engineer (CRE) and Certified Energy Manager (CEM), holding advanced degrees in business administration and environmental sustainability.